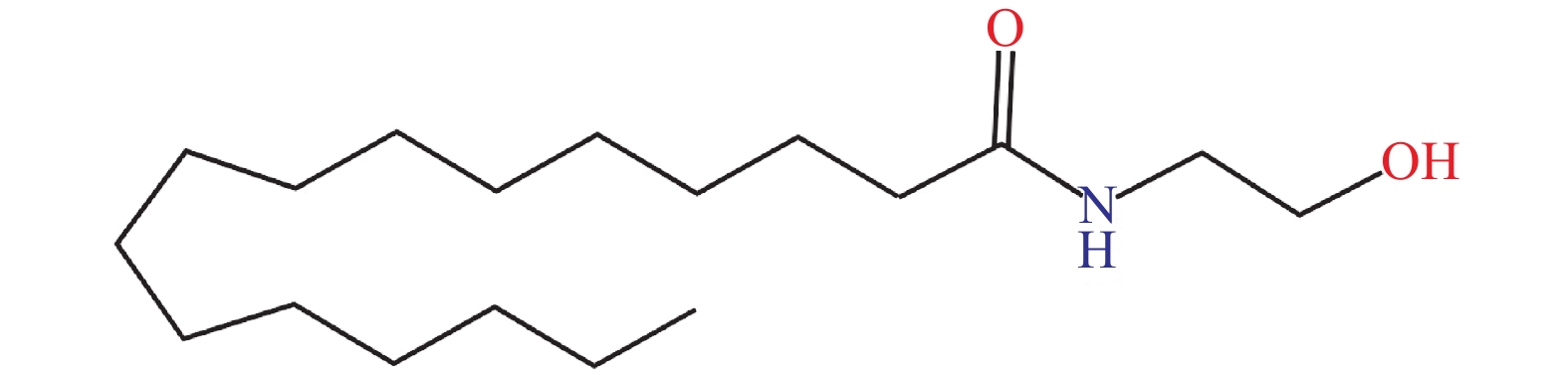
Citation: | Debasis Basu. Palmitoylethanolamide, an endogenous fatty acid amide, and its pleiotropic health benefits: A narrative review[J]. The Journal of Biomedical Research. DOI: 10.7555/JBR.38.20240053 |
Unproofed Manuscript: The manuscript has been professionally copyedited and typeset to confirm the JBR’s formatting, but still needs proofreading by the corresponding author to ensure accuracy and correct any potential errors introduced during the editing process. It will be replaced by the online publication version.
The global nutritional transition has led to high frequency and severity of chronic degenerative diseases worldwide, primarily driven by chronic inflammatory stress. At the mealtimes, various pharmaceutical products aim to prevent such an inflammatory stress, they usually cause various systemic side effects. Therefore, supplementation of natural and safe ingredients is a great strategy to reduce the risk and severity of inflammatory stress-related diseases. As a result, palmitoylethanolamide (PEA), an endocannabinoid-like mediator, has been extensively studied for its myriad of actions, including anti-inflammatory, anti-microbial, immunostimulatory, neuroprotective, and pain-reducing effects with high tolerability and safety of PEA in animals and humans. Because of the multiple molecular targets and mechanisms of action, PEA has shown therapeutic benefits in various diseases, including neurological, psychiatric, ophthalmic, metabolic, oncological, renal, hepatic, immunological, rheumatological, and gastrointestinal conditions. The current review highlights the roles and functions of PEA in various physiological and pathological conditions, further supporting the use of PEA as an important dietary agent.
Dietary nutrition plays a pivotal role in providing energy and essential building materials for maintaining optimal body health. Recent research has highlighted molecular mechanistic roles of nutrition in various biological activities and body functions[1]. However, the change of human dietary patterns from natural, nutritional diets to the processed, nutritionally deficient diets has created a "nutritional pandemic", which is considered one of the major risk factors responsible for various chronic disease conditions[2,3]. Concurrently, the change in lifestyle, including increased automated machinery and sedentary behaviors, and environmental factors, such as rising levels of pollutants, further potentiated the negative effect of this nutritional transition, further increasing conditions like obesity, dyslipidemia, metabolic syndrome (MetSyn), cardiovascular diseases, bone and joint complications, neurological disorders, oncologic diseases, among others[4,5]. Recent study has also underscored the potential role of nutrition on the gene expression level (termed nutrigenomics) and the subsequent role of the genome on the nutritional requirements (termed as nutrigenetics), potentially linking the nutritional pandemic to hereditary disease conditions[6].
The rise in chronic diseases subsequently increases the consumption of pharmaceutical medicines either as prophylactic therapy, treatment options, or maintenance of body health[7,8]. Multiple medicine use (polypharmacy) is also associated with other risks, including drug resistance, tolerance, side effects, and increased financial burdens[8,9]. These challenges underscore the need for alternative therapeutic approach that minimize adverse effects and costs, while maximizing efficacy. Natural food derivatives and constituents offer promising avenues for managing chronic diseases and enhancing overall health[10]. Among these, palmitoylethanolamide (PEA), an endocannabinoid-like mediator, has garnered attention for its multifaceted therapeutic properties. The endocannabinoid system, comprising receptors (mainly CB1 and CB2), the endogenous ligands (endocannabinoids), and degrading enzymes, plays a pivotal role in various physiological processes[10].
The bioactive lipid mediator PEA (chemical structure shown in Fig. 1) is a member of the N-acyl-ethanolamine (NAE) fatty acid amide family and resembles endocannabinoids. PEA is synthesized within the lipid bilayer 'on demand’ and is present in almost all tissues of the body[10]. PEA is typically elevated in disease states as a pro-homeostatic safeguarding defense response against cellular damage[11]. Based on various molecular studies, PEA is confirmed to have a myriad of actions including strong anti-inflammatory and pain-relieving effects, anti-convulsive effects, antimicrobial effects, antiepileptic, immunomodulatory, and neuroprotective effects as well[10]. Because of the multiple mechanisms of action, PEA may provide therapeutic benefits in many diseases of various body systems like neurology, psychiatry, ocular, metabolic, oncology, renal, hepatic, immune, joint, and gut (Fig. 2).
PEA is naturally present in various food sources, including soy lecithin, roasted coffee, black-eyed peas, apples, lentils, and potatoes, albeit in small quantities. It is also found in human milk. However, the concentrations in these sources are significantly lower than the therapeutic doses used in clinical trials, making it impractical to rely on dietary intake alone to achieve similar effects[12]. The supplementation of PEA is a feasible therapeutic approach to increase the systemic and tissue level of PEA and restore the body's normal homeostasis as the endogenous PEA level is generally reduced and becomes insufficient to combat the chronic allostatic load as observed in various chronic inflammatory disorders. Numerous nutraceuticals, health, and dietary supplements are marketed in various countries that contain PEA as the major ingredient[10].
Despite the fact that PEA has a long history of applications in various conditions, very few studies can be traced that presents the pharmacokinetic profile of oral PEA supplementation. As PEA is highly lipophilic in nature, few works have described PEA’s solubility as “trying to dissolve stones”, making its oral absorption profile highly dependent on its molecular size[12]. Due to lack of any dedicated study, the pharmacokinetic profile of PEA is highly theoretical that needs clinical validation. Other than oral route of administration, a study involving rabbits was conducted to evaluate the ocular pharmacokinetic profile of PEA after topical administration[13]. It was observed that topical ocular administration of PEA based in a nano-lipid carrier system led to the detection of PEA in the rabbit retina within 180 minutes after administration, while the topical instillation of PEA aqueous suspension led to very minor detectable quantity in the lens and vitreous humour and not in the retina[13]. Such observations highlight the fact that PEA’s absorption is highly dependent on its molecular size during administration, and hence various micronized and nano-sized PEA formulations have been developed with the aim to improve PEA absorption profile[12]. To support this assumption, an experimental pharmacokinetic study was conducted that showed significantly better absorption profile of um-PEA compared to conventional PEA formulation, confirming the influence of particle size on PEA’s absorption profile[14].
Following absorption, the next step involves the distribution profile in pharmacokinetics. By default, PEA have a high volume of distribution due to its lipophilic nature and it is well-distributed in various regions of the body, as orally administered PEA was able to reach in the hypothalamus region of the brain as well[12].
Apart from absorption and distribution, various studies have evaluated the pathways by which PEA is metabolized in the body. PEA is metabolized primarily through enzymatic pathways in the body, which involves hydrolysis. Fatty Acid Amide Hydrolase (FAAH) is the primary enzyme responsible for the hydrolysis of PEA. It breaks down PEA into palmitic acid and ethanolamine. FAAH is widely distributed in tissues, particularly in the brain and liver. Another enzyme involved in the hydrolysis of PEA, N-Acylethanolamine Acid Amidase (NAAA), is more selective for PEA and is primarily located in macrophages and other immune cells. It also converts PEA into palmitic acid and ethanolamine. Further the palmitic acid can get incorporated into phospholipid or get oxidized by beta oxidation[12].
While the end-products of PEA’s metabolism is confirmed to be palmitic acid and ethanolamine, very less can be established regarding the excretion profile of PEA based on the current available literature. While certain literature cites PEA’s excretion via renal pathways, this needs to be verified by undertaking well-designed studies[12].
A balanced diet and nutrition are major determinants that affect the endocannabinoid system. Simultaneously, changes in the endocannabinoid system are thought to have a crucial effect on appetite, eating behavior, and overall energy balance[15]. Various theories have found a link between dietary nutrition (mainly fatty acids) and endocannabinoid levels[16]. Anandamide (AEA) and 2-Arachidonoylglycerol (2-AG) are the most widely studied endocannabinoids and as they both are derived from a polyunsaturated fatty acid (arachidonic acid), it is very plausible that the dietary fatty acid content might have a potential effect on the endocannabinoid synthesis pathway[16,17]. In a recent clinical study involving participants with habitual sedentary lifestyles, the consumption of a Mediterranean diet significantly altered endocannabinoid levels by positively altering the gut microbiome and increasing the level of Akkermansia muciniphila, a mucin-degrading probiotic[18]. In the same study, it was observed that participants with different baseline endocannabinoid levels had different effects of the Mediterranean diet: the participants with low plasma oleoylethanolamide (OEA)/PEA levels had improved insulin resistance, while participants with high plasma OEA/PEA levels showed a significant reduction in systemic inflammation[18]. Likewise, a clinical study involving binge alcohol consumers showed that acute alcohol exposure is associated with alteration in serum fatty acid derivatives, including endocannabinoid levels[19]. This evidence suggests that dietary nutrition may significantly modulate the endocannabinoids level and endocannabinoid system activity by altering the molecular signaling pathways and the gut microbiome.
Similarly, the endocannabinoid system alters appetite, food intake, and energy metabolism by initiating complex signaling pathways and altering the level of orexigenic and anorexigenic mediators, including leptin and ghrelin[15,16,20]. The presence of CB1 receptors on vagal nerve neurons suggests a potential role of endocannabinoids in the cholecystokinin signaling pathway, thereby modulating the brain-derived appetite-controlling activity[16,20]. Circulating endocannabinoids has a differential effect on the adipose tissue and hepatocytes as well, ultimately modulating the levels of free fatty acids in the systemic circulation and the fate of energy metabolism in the body[15,16].
As the endocannabinoid system have influential role in various pathological conditions, research have also begun to identify endogenous and exogenous (natural and synthetic) compounds that may alter the activity of the endocannabinoid system to provide therapeutic benefits[21]. Various phytocannabinoids (including delta-9-tetrahydrocannabinol (THC), cannabidiol, and related compounds), synthetic cannabinoids (including dronabinol and nabilone), and certain agents that are currently under evaluation for their safety and clinical efficacy have been developed to stimulate the endocannabinoid system and provide therapeutic effects in different pathological conditions[21].
"Metabolic conditions" is an umbrella term used to describe various conditions in which there is an alteration in one or more metabolic pathways that ultimately impairs normal metabolism[22]. Metabolic disorders negatively alter the body's normal signaling pathways as well as the processing and distribution of macronutrients, including fats, carbs, and proteins. Obesity is a chronic metabolic disorder characterized by excessive growth of adipose tissue, as well as the accumulation of fats in non-adipose tissues. Obesity is usually accompanied by dyslipidemia, which increases cholesterol and fatty acid levels. Additionally, insulin resistance and hypertension are also commonly observed in obese individuals[23]. As the endocannabinoid system have a precise role in energy and metabolic homeostasis, abnormality in the endocannabinoid system plays a central role in the initiation and progression of obesity and MetSyn, maybe by altering appetite and energy balance, increasing lipid production and fat accumulation, impairing glucose and insulin production leading to insulin resistance, and altering the gut microbiome activity[24,25]. PEA might be a potential therapy that can be explored for the dietary management of MetSyn conditions[26].
The supplementation of umPEA increased the conversion of white adipose tissue to beige adipose tissue, along with increased thermogenic markers and leptin signaling in high-fat diet-induced obese mice, by activating peroxisome proliferator-activated receptor-alpha (PPAR-α)[27]. umPEA altered energy homeostasis, prevented fat accumulation, and modulated adipocyte differentiation, suggesting its potential role in obesity[27]. A clinical study confirmed the hypothesis of altered endocannabinoid system activity in obesity and metabolic conditions, showing significant alterations in the levels of endocannabinoids in insulin-resistant obese post-menopausal women compared with insulin-sensitive obese post-menopausal women[28]. The study also indicated that high serum PEA levels might be associated with weight loss by promoting a positive inflammatory environment[28]. Because gut microbiome dysfunction plays a crucial role in the progression of a systemic inflammatory state and obesity[25], umPEA was found to improve the gut microbiome by enhancing PPAR-α activity and associated tryptophan metabolism[29].
PEA’s role in metabolic health extends to liver function, one of the most important metabolic organs in the body. The presence of EC receptors on hepatic cells makes it plausible to assume that PEA administration may directly or indirectly (by entourage effect) modulate the hepatic metabolism. In a preliminary experimental study, PEA therapy significantly altered level of fatty acid, glucose, amino acid, and hepatic growth rate, demonstrating the positive role of PEA in hepatic health[30]. Fatty liver disease is a metabolic condition associated with increased intrahepatic accumulation of fatty acids which activates a reactive chain within the liver, ultimately leading to increased oxidative stress and inflammation that damages hepatic cells and leads to non-alcoholic steatohepatitis (NASH). The sustained hepatic inflammation further activates hepatic stellate cells, thereby progressing NASH to hepatic fibrosis and further to hepatocellular carcinoma and end-stage liver disease state[31]. PEA administration is observed to reduce the activation of hepatic Kupffer and stellate cells in an in-vitro study using hepatic stellate cell line, while in carbon tetrachloride-induced liver fibrosis animal model, PEA therapy significantly reduced hepatic type-I collagen deposition and overall hepatic fibrosis rate[32]. Similarly, PEA therapy is associated with a significant reduction in hepatic lipid accumulation and insulin resistance, while improvement in total energy expenditure[33]. Although PPAR-α is the principle target for PEA’s activity, some studies have highlighted the PPAR-α independent hepatoprotective action as well. In an experimental NASH study, umPEA supplementation improved the expression of PPAR-α and the phosphorylation level of adenosine monophosphate (AMP)-activated protein kinase (AMPK), while reduced the expression of acetyl-CoA carboxylase-1 (ACC1) and CD36 in hepatic cells[34]. ACC1 and CD36 have important role in hepatic lipogenesis and fatty acid infiltration, as well as in hepatic cancer development and progression[35,36]. Reduction of ACC1 and CD36 expression by umPEA was associated with a significant reduction in inflammatory mediators, oxidative stress, and improved hepatic antioxidant potential, which in turn improved liver functioning, prevented lipid accumulation, and significantly prevented the pathological progression of hepatic fibrosis[34]. Such positive results highlight the hepatoprotective activity of PEA in fatty liver and NASH condition.
PEA also plays a crucial role in gastrointestinal health by interacting with the gut microbiome, which is essential for maintaining the normal functioning of the gastrointestinal system and the endocannabinoid environment[37]. In an in vitro study, the addition of fatty acid endocannabinoids to a gut microbiome-like culture significantly increased the beneficial microbes and reduced the pathogenic microbes[37]. As the fatty acid endocannabinoids share structural similarity with long-chain fatty acids (LCFAs), which are the anti-microbial agents produced by gut microbes, it can be postulated that the gut microbiome consumes the fatty acid endocannabinoids to form and release LCFAs. Additionally, being a part of the cellular membrane, fatty acid endocannabinoids may also improve the membrane stability of the gut microbiome and the intestinal epithelial cells, thereby improving gut barrier integrity and overall gut health[37]. PEA is reported to prevent intestinal damage and therefore prevent the absorption of lactulose and mannitol[38]. These observations highlight the fact that a positive endocannabinoid signaling is essential for intestinal health and exogenous PEA supplementation may improve overall gut health.
The endocannabinoid system is widely distributed in the cardiovascular system (CVS) with the receptors present in the cardiac muscle, endothelial and smooth muscles, and on the pre-synaptic sympathetic nerve terminals that innervate the CVS[39]. The positive role of PEA (either as a single umPEA ingredient or in combination with other ingredients like polydatin and baicalein) has been evaluated in various experimental vascular injury and myocardial ischemic animal model studies[40–42]. PEA supplementation was associated with a significant reduction in the vascular injury-induced inflammatory process, reperfusion-associated myocardial injury, cellular apoptosis, and the expression and levels of immune adhesion molecules[40–42]. Similarly, an experimental lipopolysaccharide-induced thrombosis animal model study showed that the umPEA supplementation reduced the blood coagulation markers and prevented the deposition of fibrin, by reducing the proinflammatory biomarkers[43]. Hypertension is one of the major cardiovascular disease conditions leading to a severe impact on the health of individuals. As a potent PPAR-α agonist, PEA has been demonstrated to improve blood pressure and prevent hypertension-related kidney damage in spontaneously hypertensive rats[44]. Additionally, PEA supplementation was associated with an improvement in endothelium-derived hyperpolarizing factors that resulted in the vasodilation of arteries[44]. In the same study, it was observed that PEA supplementation significantly reduced the expression of angiotensin receptor 1 and angiotensin-converting enzyme, which indicated that PEA may prevent hypertension by acting on the angiotensin-signaling pathway as well[44]. Additionally, by interacting with the vascular CB1, transient receptor potential vanilloid-1, and probably G protein-coupled receptor 55 receptors, PEA is capable of modulating the sympathetic stimulation-related vascular contractile responses as well, which further supports the anti-hypertensive potential of PEA[45].
The kidneys are one of the major organs involved in the elimination of metabolism by-products and toxins, regulating body volume status, maintaining electrolyte balance and normal body homeostasis[46]. With the constant rise in the prevalence of acute kidney disease and chronic kidney disease (CKD), the search for new therapies to improve renal health has also increased in recent decades. The cannabinoid receptors have demonstrated their role in maintaining normal renal hemodynamics and overall health, while the alterations in renal endocannabinoid system activity is associated with various renal complications[46]. In a renal ischemia/reperfusion (I/R)-induced renal injury animal model, PEA supplementation significantly attenuated the renal dysfunction rate. Similarly, in an experimental study involving mice subjected to renal I/R injury, supplementation of PEA along with silymarin was found to significantly attenuate renal dysfunction and histological damage compared with silymarin alone supplementation[47]. PEA potentially alters the NF-κB signalling, thereby reducing the expression of pro-inflammatory cytokines and improving the expression of antioxidants, thereby reducing oxidative stress and a renal-protective effect[47]. Similarly, in a study involving hypertensive rats, PEA therapy reduced the expression of cytochrome P450 (CYP) 4A, CYP2C23, and soluble epoxide hydrolase in renal tissues, indicating reduced inflammation and a renal-protective effects[48]. Additionally, PEA reduced the expression of key reactive oxygen species (ROS) and reactive nitrogen species (RNS) synthesizing enzymes, including renal nicotinamide adenine dinucleotide phosphate hydrogen (NADPH) oxidase and inducible nitric oxide synthase (iNOS), thus reducing oxidative stress and maintaining a normal renal homeostatic balance[47,48].
Diabetic nephropathy (DN) is one of the most common diabetic renal complications which involves chronic systemic low-grade inflammation and oxidative stress which can lead to CKD[49,50]. In an I/R-induced renal damage animal model, PEA therapy prevented the renal dysfunction by activating PPAR-α, and thereby reducing the degree of inflammation and renal damage[51]. Similarly in hypertensive rats, PEA therapy improved the angiotensin-1/angiotensin-2 balance and the renin-angiotensin-aldosterone system activity, thereby improving blood volume and blood pressure and overall renal hemodynamics[51]. In a contrast-induced nephropathy animal model, umPEA therapy significantly reduced renal inflammation, thereby preventing the glomerular dysfunction and level of renal parenchymal damage[52]. This evidence highlights the potential of PEA in preventing the progression of CKD by improving the overall inflammatory environment.
Stroke is a medical condition associated with the blockage of blood supply to certain areas of the brain, which may be caused by a vascular block (ischemic stroke) or the sudden rupture of a cranial blood vessel, leading to hemorrhage and reduced cranial blood supply (hemorrhagic stroke)[53]. Ischemic stroke is associated with a series of events that lead to the disruption of blood-brain barrier (BBB) integrity and cerebral inflammation[54]. In an experimental middle cerebral artery occlusion-induced stroke animal model, PEA therapy significantly prevented brain histological and cellular damage by reducing the level of inflammatory cytokines[55]. Also, by activating PPAR-α, PEA therapy prevented the expression of phosphorylated Jun kinase (pJNK) and nuclear factor kappa-B (NF-κB), thereby preventing cellular apoptosis, ischemia-induced cerebral damage, and neurobehavioral alterations[55]. Similarly, in a cerebral ischemia and reperfusion-induced stroke animal model, PEA therapy was associated with decreased inflammatory cytokine expression level, volume of infarction, cerebral edema level, and improved neurological function[56]. PEA was found to prevent early BBB disruption events by inhibiting the activation of Rho-associated protein kinase (ROCK) and myosin light chain kinase (MLC) signaling, a BBB-protective activity that is independent of PPAR-α signaling[56]. Molecular studies have identified that ROCK/MLC signaling causes disruption of BBB integrity by increasing expression of pro-inflammatory cytokines and formation of cellular adhesion complexes[57]. Hence, PEA mediated inhibition of ROCK/MLC signaling may open a potential new therapeutic door to prevent BBB disruption during ischemic stroke[56]. Stroke is associated with increased neuroinflammation, which further activates a cascade of inflammatory signaling pathways, further damaging cerebral tissues. In an ischemia-induced cerebral injury model, PEA activated the PPAR-α and reduced neuroinflammation by shifting the inflammatory M1-macrophage to an anti-inflammatory M2 phenotype[58]. Similar neuroprotective effect was observed in an experimental middle cerebral artery occlusion-induced brain injury model by umPEA with luteolin (PEALut)[59]. To support the experimental findings, a clinical trial evaluated the effectiveness of PEALut in patients with acute ischemic stroke who were undergoing thrombolytic treatment[60]. In the study, the patients receiving PEA supplement along with standard therapy showed significantly better recovery and cognitive improvement compared with standard group patients, which concludes that PEA is a safe and effective therapy that may reduce the severity of neuroinflammation and improve the effectiveness of standard therapies for the better management of acute ischemic stroke condition[60].
Migraine is a condition of recurrent impulsive headaches of unknown origin, with various evidence suggesting neuroinflammation to play an important role[61]. The trigeminovascular system (TS) is one of the major systems identified to be involved in migraine in which the sequential activation of TS causes release of various neurogenic peptides from trigeminal endings into the meningeal vessels, leading to release of various inflammatory mediators from the meninges[62]. Prolonged inflammation reduces nociceptor activation threshold, leading to central and peripheral sensitization[62]. Some studies have identified that the level of AEA, one of the major endocannabinoid lipids, is reduced in the serum and cerebrospinal fluid of migraine patients highlighting that alteration in the endocannabinoid system may be involved in migraine, and therapies targeting the endocannabinoid system activity may be beneficial in migraine[62]. In a study involving children with recurrent migraine without aura, three months of umPEA therapy significantly reduced the number and severity of migraine attacks supporting the use of PEA as prophylactic therapy for migraines[63]. Similarly, in a study including subjects with migraine with aura, 90 days of umPEA therapy along with non-steroidal anti-inflammatory drugs (NSAIDs) showed greater relief from migraine pain compared with subjects treated with NSAIDs alone, further supporting the role of PEA for migraine prophylaxis and treatment[64]. In another open-label study involving patients with migraine with or without aura, supplementation of a PEA-containing nutraceutical reduced the number and intensity of migraine pain attacks and the use of analgesic medications for pain relief[65]. While PEA is proven to improve the level of AEA and show neuroprotective, anti-inflammatory and analgesic activity, more studies are required to identify the exact mechanistic role of PEA in migraine condition[62,66].
Mild cognitive impairment (MCI) is a cognitive impairment disorder that does not significantly affect the normal daily life activities of individual but considered as the pre-symptomatic stage of dementia. MCI is divided into two types, amnestic-MCI in which only memory is affected, while non-amnestic-MCI in which one or more cognitive domains other than memory are affected[67]. The potential of PEA in MCI has been demonstrated in a case-study in which an elderly female patient with amnestic-MCI showed significant improvement in cognitive performance as well as improved perfusion rate (as evaluated using single- photon emission computed tomography (SPECT) analysis) after nine months PEALut therapy[68]. These observations, being preliminary, highlights the positive neuroprotective efficacy of PEA partly by resolving neuroinflammation via PPAR-α agonistic activity[68].
Alzheimer's disease (AD) is a neurodegenerative condition that involves brain neuronal atrophy leading to progressive memory loss, and cognitive decline. The formation of amyloid beta (Aß) plaques on the outer surface of the brain and hyperphosphorylated tau protein within neurons are the most common hallmarks of AD[67]. Various experimental studies have showed that PEA may counteract neuroinflammation and neuronal damage by activating PPAR-α, while protects neurons by preventing Aß-induced reactive gliosis[67]. In Aß-induced neuronal damage animal model, PEA therapy significantly reduced neuronal damage rate and associated cognitive, learning, and memory impairment[67]. PEA by activating PPAR-α may attenuate the neuroinflammation and neuronal atrophy observed in AD. In another in vitro study, the addition of Aß in astrocyte cell line prevented the release of astrocyte-induced growth factor, reduced the oligodendrocytes maturation rate, and increased inflammatory cytokines release, while PEALut therapy counteracted this Aß-induced astrocyte reactivity, probably by activating PPAR-α[69]. This result provides strong evidence for the clinical evaluation of PEA supplements in AD patients.
Parkinson's disease (PD) is an age-related neurodegenerative disease associated with the loss of dopaminergic neurons in the nigrostriatal pathway leading to the characteristic physical symptoms of excessive shaking, muscle stiffness, and walking and balance coordination impairment[67]. Various studies have suggested an important role of neuroinflammation in PD pathogenesis[70]. In a 1-methyl-4-phenyl-1,2,3,6-tetrahydropyridine-induced neurotoxicity PD animal model, PEA significantly prevented the loss of brain dopaminergic neurons and development of motor deficits, while promoted neurogenesis, probably by activating PPAR-α and providing anti-inflammatory effect[67]. Similarly, the motor disturbances caused by unilateral intra-striatal 6-hydroxydopamine injection were attenuated following PEA administration by preventing neuroinflammation-induced neurotoxicity and cell death[67]. In PD patients, the supplementation of umPEA and PEALut was associated with a significant reduction in motor and non-motor symptoms, improvement in dyskinesia of the legs and trunk, and a significant reduction in the onset of camptocormia, a common spine deformity observed in PD patients[71,72]. Such neuroprotective role of PEA supports its use in clinical settings for better PD management.
Huntington's disease (HD) is a rare autosomal dominant disorder characterized by a mutation in the huntingtin gene on chromosome 4 causing overproduction of the mutant huntingtin (m-Htt) protein, which aggregates in brain regions leading to neuronal dysfunction and degeneration[73]. HD leads to loss of GABAergic medium spiny neurons and astrogliosis, which leads to the characteristic HD motor disturbances (known as chorea), dementia, psychiatric symptoms, mood swings, and early mortality rates[67,74]. Among various determinants, neuroinflammation plays an important role in the HD disease progression and symptoms development[75]. In an experimental study involving transgenic mice with HD, the brain level of major endocannabinoids, including PEA, were significantly reduced in the striatum region, highlighting the fact that the endocannabinoid system is crucial for the normal functioning of the brain, and its abnormality can lead to neuroinflammation and subsequent neuronal damage and atrophy in HD[67].
Neuropathy is a type of functional somatic syndrome characterized by persistent chronic pain but without any overt sign of physical or structural bodily injury or defects. Various factors, including tissue injury, infections, disease conditions, and stress, can cause peripheral nerve damage and lead to inflammatory state which in turn activates the nociceptors and cause painful sensations[76]. The prolonged inflammation during neuropathy reduces the nociceptors threshold and sensitivity, leading to increased pain perception, a condition known as pain sensitization[76]. PEA is considered as a disease-modifying agent in neuropathy due to its anti-nociceptive and anti-inflammatory properties[77]. In chronic constriction injury-induced neuropathy, PEA supplementation significantly reduced pain and improved neuronal health, by activating PPAR-α and reducing the inflammatory cascade[77]. Data from clinical studies also show that PEA along with other standard therapies such as gabapentinoids, opioid analgesics, transdermal fentanyl and buprenorphine, and paracetamol has shown clinically meaningful reduction in pain in patients with various chronic painful conditions, including radiculopathy[78]. PEA, by activating PPAR-α, suppresses the nociceptive signaling, which has demonstrated its efficacy in reducing pain and improving the quality of life of more than
Amyotrophic lateral sclerosis (ALS) is a CNS neurodegenerative condition majorly caused by mutations in the TARDBP gene, leading to destruction of TAR DNA-binding protein-43 (TDP-43) and aggregation on the motor neurons, which present as a motor neuron dysfunction and leads to progressive weakness of voluntary muscles involved in limb movement, difficulty in swallowing, speaking, and respiratory activity, along with cognitive changes like poor working memory, lack of interest, irritability, abnormal eating behavior, and altered language fluency[79]. The neurodegeneration in ALS is associated with significant spinal inflammation mediated mainly by mast cells and microglial cells[67]. Based on this inference, two clinical studies observed the clinical benefits of PEA therapy in ALS patients reporting improvement in muscle tone, respiratory, and motor functioning, by controlling the overactivity of mast and microglial cells and reducing neuroinflammation[67].
Multiple sclerosis (MS) is an autoimmune demyelinating disease associated with progressive immune-mediated attacks on the neuronal myelin sheath and inflammation, which further recruits more immune cells in the brain causing further damage to the myelin sheath[80]. The beneficial anti-inflammatory effect of PEA has been evaluated in an experimental autoimmune encephalomyelitis MS animal model in which the intraperitoneal administration of PEA was associated with a reduction in the severity of inflammation, leading to reduced demyelination rate, neuronal damage, and neurobehavioral impairment[67]. In a clinical study, umPEA therapy significantly reduced serum cytokine levels and improved overall quality of life in MS patients suggesting positive role of PEA in MS management[81].
Fibromyalgia (FM) is a condition associated with chronic widespread pain and tenderness in deep tissues and muscles, fatigue, and reduced muscle strength[82]. Numerous evidence have suggested that chronic inflammation plays an important role in increasing nociceptive sensations and reducing pain threshold in FM[83]. PEA is able to modulate inflammation by activating PPAR-α and reducing the activity of non-neuronal cells (mast and glial cells), thereby reducing painful sensations. In a clinical study involving FM patients previously treated with duloxetine + pregabalin (DuoPre), umPEA add-on therapy showed greater reduction in pain and FM tender points compared with DuoPre alone therapy[84]. In another similar clinical study, FM patients previously under DuoPre therapy were randomized to receive PEA and acetyl-L-carnitine combination along with the DuoPre therapy or to continue the DuoPre therapy only. The addition of PEA supplement showed significantly greater reduction in pain and improvement in the quality of life compared with DuoPre therapy alone, which supports the hypothesis that PEA, by reducing inflammation, provides an analgesic effect and can also improve the analgesic property of standard therapeutic regimens[85]. Another clinical study showed positive reduction in pain and improved overall quality of life of FM patients by umPEA therapy[86].
The basic pharmacology and functioning of the retina show high similarity with CNS neuronal cells, starting from receiving signals to converting them into electrical impulses[87]. Hence, as the endocannabinoid system have crucial role in maintaining CNS health, it is plausible to say that the endocannabinoid system plays a crucial role in retinal and overall ophthalmic health as well[87]. Degenerative retinal disease is a condition associated with progressive retinal damage leading to retinopathy and loss of vision. Among the various risk factors, chronic inflammation in the retinal area plays a central role in majorly all types of retinopathies including diabetic retinopathy (DR), glaucoma, and age-related macular degeneration (AMD)[88].
Glaucoma is a condition in which the intraocular pressure (IOP) is progressively increased which damages the retina and can lead to blindness[89]. Among the other determinants, certain evidence have supported the role of retinal inflammation in increasing IOP in glaucoma[89]. The possible retinoprotective effect of PEA has been explored in various experimental studies[89]. In a study involving assessment of human eye tissues obtained from normal and glaucomatous patient donors, the level of PEA was significantly reduced in the ciliary body, which raised the possibility that PEA is important for maintaining normal IOP level by regulating the normal functioning of the ciliary body[90]. To support this hypothesis, various clinical studies have showed that PEA therapy effectively reduces IOP and improves associated visual field activity, tear properties, endothelial functioning, and retinal functionality[91–94].
Diabetic retinopathy is the major ophthalmic complication of diabetes affecting around 30%–40% of diabetics[95]. As PEA have an anti-inflammatory, retinoprotective, and antioxidant potential, its therapy in streptozotocin-induced diabetic rats significantly prevented the retinal damage and preserved the blood-retinal barrier by reducing inflammation level[96]. To support these evidence, clinical studies may be conducted to support the use of PEA in DR condition.
Age-related macular degeneration is a progressive and irreversible damage of the retina and associated tissues[97]. AMD is of two types, namely non-neovascular AMD ('dry' type) and neovascular AMD ('wet' type)[97]. Among the various determinants of this complex condition, inflammation is thought to be one of the main factors involved in the pathogenesis of AMD, as it is observed that ocular inflammation causes choroidal neovascularization and ocular tissues atrophy[98]. In an oxygen-induced retinopathy and very-low-density lipoprotein receptor knockout (Vldlr−/−) mouse model of retinopathy, PEA therapy significantly prevented the profibrotic changes of retinal tissues, and by activating PPAR-α, reduced inflammation and the development of Müller gliosis, providing preliminary evidence that PEA provides a positive effect in preventing retinal damage observed in AMD condition[99]. These evidence suggest the potential role of PEA in ophthalmic health which needs to be considered in future as a potential therapeutic option.
Bone is a dynamic organ that is constantly subjected to formation and breakdown for maintaining the normal homeostasis of the bone and the body. Research have identified peculiar role of EC system maintaining bone health[100]. A supplement containing dispersible PEA has shown a significant reduction in both morning and evening joint pain[101]. Similarly in osteoarthritis condition, PEA therapy showed a significant reduction in in the level of pro-inflammatory mediators like leukotriene B4, tumor necrosis factor-α, interleukin-1ß, prostaglandin E2, and cartilage-degrading enzymes, including matrix metalloproteinases−2, −3, −9, and −13, which lead to reduction in joint swelling and cartilage destruction[102]. Such data supports the beneficial anti-inflammatory role of PEA in maintaining optimal bone and joint health.
First described in 1970s, when the growth leukemia cells was reduced after treatment with THC, the active constituent of the medicinal plant Cannabis sativa[103], various studies have evaluated the anti-neoplastic potential of endogenous and plant-derived cannabinoids, including PEA.
Breast cancer is the most common type of cancer in the female population. The research on the use of PEA in breast cancer condition is still in its infancy. In an in vitro study using human breast cancer cell line, PEA treatment inhibited the activity of fatty acid amide hydrolase, the enzyme responsible for the degradation of AEA, which in return potentiated the anti-neoplastic effect of AEA by increasing the activation of CB-receptors and caused a reduction in the expression and signaling of nerve growth factor/TrkA activity, a signaling pathway causing increased activation of the Ras/MAPK pathway, leading to increased proliferation, invasion, and metastasis of breast cancer cells[104,105].
Colorectal cancer (CRC) is one of the most common cancers globally and among its various determinants, alterations in energy homeostasis has been associated with the development and/or progression of CRC[106]. PEA, being a modulator of overall energy homeostasis, has shown to upregulate the cyclin B1/cyclin-dependent kinase-1 pathway, thereby resulting in cell cycle arrest and initiated DNA fragmentation in a pre-clinical murine model of colon cancer, probably by activating PPAR-α and GPR-55[107]. This action was supported with a significant reduction in the number of preneoplastic lesions and tumors in PEA-supplemented animals, which provides a new understanding of the role of PPAR-α and GPR-55 in the cell cycle and the anti-neoplastic potential of PEA[107].
Cervical cancer, predominantly by human papillomavirus infection, is the third leading malignancy in the female population[108]. Among the various molecular pathways, the ubiquitin-proteasome pathway (UPP)-mediated degradation of cell cycle regulatory molecules is one of the major pathways involved in the pathogenesis of cervical cancer[109]. UPP is a protein degradation pathway that involves the ubiquitin protein-mediated degradation of target protein by 20S-proteasome complex[110]. While the UPP system plays an essential role in overall protein turnover rate, its uncontrolled activation and the destruction of cell-cycle regulatory proteins (namely cyclin, cyclin-dependent kinases, and cyclin-dependent kinase inhibitors) can lead to uncontrolled cellular proliferation and ultimately cancer[110]. In a preliminary in vitro study using human cervical cancer (HeLa) cells, the exogenous addition of PEA significantly inhibited the proteasome complex and increased the Caspase-3 activity, thereby highlighting the anti-neoplastic efficacy of PEA[111]. Such positive results are required to be validated in further experimental pre-clinical studies and confirmed in clinical studies.
The immune system is an important defence system of the body. PEA first appeared on the market in the 1960s for the prophylactic treatment of influenza and common cold[10]. PEA by activating PPAR-α, regulates macrophages and determines the degranulatory fate of mast cells. Additionally, by the entourage effect, PEA may modulate the non-specific innate immune response against various bacterial and viral pathogens[10]. Based on these actions, PEA shows a broad-spectrum anti-viral and anti-bacterial activity[10]. In patients with severe acute respiratory syndrome coronavirus-2 (SARS-CoV2), PEA therapy significantly improved the olfactory response, probably by the anti-inflammatory activity[112].
PEA has been shown to be both safe and effective in alleviating pain and enhancing the quality of life (QoL) in over 6,000 patients with chronic pain conditions. Studies involving these patients have consistently demonstrated significant reductions in pain levels, along with notable improvements in their overall well-being and daily functioning. This evidence supports the use of PEA as a reliable option for managing chronic pain and improving the quality of life in affected individuals[78]. The safety of PEA is further illustrated in Fig. 3 [113,114].
Despite the advancement in the modern medicinal science, the role of balanced nutrition is and will always be pivotal for maintaining healthy disease-free life. But, the current state of the nutrition epidemic is increasing in alarming rate that is responsible for various alterations in the normal physiological processes leading to chronic disease conditions. One of the physiological pathways is the endocannabinoid system which is considered to have a important role in various disease conditions. With the expansion in the methods of molecular studies, it is now well-known that dietary nutrition and endocannabinoid system have a significant bi-directional interaction with each other. Based on this inference, it can be stated that therapies that can positively alter the endocannabinoid system might have great therapeutic potential in various disease conditions. This particular hypothesis has led to the development of drug candidates that can positively modulate the activity of the endocannabinoid system. The current review article particularly highlighted the potential of palmitoylethanolamide, a "non-typical" endogenous cannabinoid, produced in the body when required in response to various stimuli. The in-depth literature review presented in this article provides substantial evidence for the potential role of palmitoylethanolamide in normal healthy conditions and its putative role in various disease conditions. But, despite the large experimental and clinical investigations undertaken with positive results, many more domains are still required to be explored to fully support the therapeutic potential of palmitoylethanolamide. The present review article has also underscored some major research need-gaps that may guide future researchers regarding the varied roles of palmitoylethanolamide and design experimental and clinical studies that might bridge these knowledge gaps.
None.
None.
CLC number: R151, Document code: A
The authors reported no conflict of interests.
[1] |
Elmadfa I, Meyer AL. Importance of food composition data to nutrition and public health[J]. Eur J Clin Nutr, 2010, 64(S3): S4–S7. doi: 10.1038/ejcn.2010.202
|
[2] |
Myles IA. Fast food fever: reviewing the impacts of the western diet on immunity[J]. Nutr J, 2014, 13: 61. doi: 10.1186/1475-2891-13-61
|
[3] |
Astrup A, Dyerberg J, Selleck M, et al. Nutrition transition and its relationship to the development of obesity and related chronic diseases[J]. Obes Rev, 2008, 9(S1): 48–52. doi: 10.1111/j.1467-789X.2007.00438.x
|
[4] |
Senapati S, Bharti N, Bhattacharya A. Modern lifestyle diseases: chronic diseases, awareness and prevention[J]. Int J Curr Res Acad Rev, 2015, 3(7): 215–223. http://www.ijcrar.com/vol-3-7/Sabyasachi%20Senapati,%20et%20al.pdf
|
[5] |
Egger G, Dixon J. Beyond obesity and lifestyle: a review of 21st century chronic disease determinants[J]. Biomed Res Int, 2014, 2014: 731685. doi: 10.1155/2014/731685
|
[6] |
Tapsell LC, Probst YC. Nutrition in the prevention of chronic diseases[M]//Simopoulos AP. Nutrition and Fitness: Cultural, Genetic and Metabolic Aspects. New York: Karger, 2008: 94–105.
|
[7] |
Ünsal A, Demir G. The prevalence of chronic disease and drug use in the elderly in central Kirsehir[J]. Turk J Geriatr, 2010, 13(4): 244–251.
|
[8] |
Rieckert A, Trampisch US, Klaaßen-Mielke R, et al. Polypharmacy in older patients with chronic diseases: a cross-sectional analysis of factors associated with excessive polypharmacy[J]. BMC Fam Pract, 2018, 19(1): 113. doi: 10.1186/s12875-018-0795-5
|
[9] |
Almodóvar AS, Nahata MC. Associations between chronic disease, polypharmacy, and medication-related problems among medicare beneficiaries[J]. J Manag Care Spec Pharm, 2019, 25(5): 573–577. doi: 10.18553/jmcp.2019.25.5.573
|
[10] |
Clayton P, Hill M, Bogoda N, et al. Palmitoylethanolamide: a natural compound for health management[J]. Int J Mol Sci, 2021, 22(10): 5305. doi: 10.3390/ijms22105305
|
[11] |
Darmani NA, Izzo AA, Degenhardt B, et al. Involvement of the cannabimimetic compound, N-palmitoyl-ethanolamine, in inflammatory and neuropathic conditions: review of the available pre-clinical data, and first human studies[J]. Neuropharmacology, 2005, 48(8): 1154–1163. doi: 10.1016/j.neuropharm.2005.01.001
|
[12] |
Rankin L, Fowler CJ. The basal pharmacology of palmitoylethanolamide[J]. Int J Mol Sci, 2020, 21(21): 7942. doi: 10.3390/ijms21217942
|
[13] |
Puglia C, Blasi P, Ostacolo C, et al. Innovative nanoparticles enhance N-palmitoylethanolamide intraocular delivery[J]. Front Pharmacol, 2018, 9: 285. doi: 10.3389/fphar.2018.00285
|
[14] |
Petrosino S, Cordaro M, Verde R, et al. Oral ultramicronized palmitoylethanolamide: plasma and tissue levels and spinal anti-hyperalgesic effect[J]. Front Pharmacol, 2018, 9: 249. doi: 10.3389/fphar.2018.00249
|
[15] |
Pagotto U, Marsicano G, Cota D, et al. The emerging role of the endocannabinoid system in endocrine regulation and energy balance[J]. Endocr Rev, 2006, 27(1): 73–100. doi: 10.1210/er.2005-0009
|
[16] |
Carr TP, Jesch ED, Brown AW. Endocannabinoids, metabolic regulation, and the role of diet[J]. Nutr Res, 2008, 28(10): 641–650. doi: 10.1016/j.nutres.2008.08.003
|
[17] |
Hansen HS, Artmann A. Endocannabinoids and nutrition[J]. J Neuroendocrinol, 2008, 20(S1): 94–99. doi: 10.1111/j.1365-2826.2008.01687.x
|
[18] |
Tagliamonte S, Laiola M, Ferracane R, et al. Mediterranean diet consumption affects the endocannabinoid system in overweight and obese subjects: possible links with gut microbiome, insulin resistance and inflammation[J]. Eur J Nutr, 2021, 60(7): 3703–3716. doi: 10.1007/s00394-021-02538-8
|
[19] |
Antón M, Rodríguez-González A, Rodríguez-Rojo IC, et al. Increased plasma oleoylethanolamide and palmitoleoylethanolamide levels correlate with inflammatory changes in alcohol binge drinkers: the case of HMGB1 in women[J]. Addict Biol, 2018, 23(6): 1242–1250. doi: 10.1111/adb.12580
|
[20] |
Watkins BA, Kim J. The endocannabinoid system: directing eating behavior and macronutrient metabolism[J]. Front Psychol, 2015, 5: 1506. doi: 10.3389/fpsyg.2014.01506/full
|
[21] |
An D, Peigneur S, Hendrickx LA, et al. Targeting cannabinoid receptors: current status and prospects of natural products[J]. Int J Mol Sci, 2020, 21(14): 5064. doi: 10.3390/ijms21145064
|
[22] |
Golden GS. Metabolic disorders[M]//Golden GS. Textbook of Pediatric Neurology. New York: Springer, 1987: 179–193.
|
[23] |
Gupta P, Tyagi S, Mukhija M, et al. Obesity: an introduction and evaluation[J]. J Adv Pharm Educ Res, 2011, 2: 125–137. https://www.speronline.com/japer/Articlefile/29.pdf
|
[24] |
Dörnyei G, Vass Z, Juhász CB, et al. Role of the endocannabinoid system in metabolic control processes and in the pathogenesis of metabolic syndrome: an update[J]. Biomedicines, 2023, 11(2): 306. doi: 10.3390/biomedicines11020306
|
[25] |
Di Marzo V, Silvestri C. Lifestyle and metabolic syndrome: contribution of the endocannabinoidome[J]. Nutrients, 2019, 11(8): 1956. doi: 10.3390/nu11081956
|
[26] |
Patil AS, Mahajan UB, Agrawal YO, et al. Plant-derived natural therapeutics targeting cannabinoid receptors in metabolic syndrome and its complications: a review[J]. Biomed Pharmacother, 2020, 132: 110889. doi: 10.1016/j.biopha.2020.110889
|
[27] |
Annunziata C, Pirozzi C, Lama A, et al. Palmitoylethanolamide promotes white-to-beige conversion and metabolic reprogramming of adipocytes: contribution of PPAR-α[J]. Pharmaceutics, 2022, 14(2): 338. doi: 10.3390/pharmaceutics14020338
|
[28] |
Abdulnour J, Yasari S, Rabasa-Lhoret R, et al. Circulating endocannabinoids in insulin sensitive vs. insulin resistant obese postmenopausal women. A MONET group study[J]. Obesity (Silver Spring), 2014, 22(1): 211–216. doi: 10.1002/oby.20498
|
[29] |
Pirozzi C, Coretti L, Opallo N, et al. Palmitoylethanolamide counteracts high-fat diet-induced gut dysfunction by reprogramming microbiota composition and affecting tryptophan metabolism[J]. Front Nutr, 2023, 10: 1143004. doi: 10.3389/fnut.2023.1143004
|
[30] |
Obermajerová H, Seifert J, Buchar E, et al. Biochemical changes in mouse liver after palmitoylethanolamide (PEA) administration[J]. Chem Biol Interact, 1973, 6(4): 219–226. doi: 10.1016/0009-2797(73)90049-5
|
[31] |
Fernando DH, Forbes JM, Angus PW, et al. Development and progression of non-alcoholic fatty liver disease: the role of advanced glycation end products[J]. Int J Mol Sci, 2019, 20(20): 5037. doi: 10.3390/ijms20205037
|
[32] |
Ohara M, Ohnishi S, Hosono H, et al. Palmitoylethanolamide ameliorates carbon tetrachloride-induced liver fibrosis in rats[J]. Front Pharmacol, 2018, 9: 709. doi: 10.3389/fphar.2018.00709
|
[33] |
Annunziata C, Lama A, Pirozzi C, et al. Palmitoylethanolamide counteracts hepatic metabolic inflexibility modulating mitochondrial function and efficiency in diet-induced obese mice[J]. FASEB J, 2020, 34(1): 350–364. doi: 10.1096/fj.201901510RR
|
[34] |
Hu J, Ying H, Yao J, et al. Micronized palmitoylethanolamide ameliorates methionine- and choline-deficient diet-induced nonalcoholic steatohepatitis via inhibiting inflammation and restoring autophagy[J]. Front Pharmacol, 2021, 12: 744483. doi: 10.3389/fphar.2021.744483
|
[35] |
Ye B, Yin L, Wang Q, et al. ACC1 is overexpressed in liver cancers and contributes to the proliferation of human hepatoma Hep G2 cells and the rat liver cell line BRL 3A[J]. Mol Med Rep, 2019, 19(5): 3431–3440. doi: 10.3892/mmr.2019.9994
|
[36] |
Zeng H, Qin H, Liao M, et al. CD36 promotes de novo lipogenesis in hepatocytes through INSIG2-dependent SREBP1 processing[J]. Mol Metab, 2022, 57: 101428. doi: 10.1016/j.molmet.2021.101428
|
[37] |
Ellermann M. Emerging mechanisms by which endocannabinoids and their derivatives modulate bacterial populations within the gut microbiome[J]. Adv Drug Alcohol Res, 2023, 3: 11359. doi: 10.3389/adar.2023.11359
|
[38] |
Couch DG, Cook H, Ortori C, et al. Palmitoylethanolamide and cannabidiol prevent inflammation-induced hyperpermeability of the human gut in vitro and in vivo-a randomized, placebo-controlled, double-blind controlled trial[J]. Inflamm Bowel Dis, 2019, 25(6): 1006–1018. doi: 10.1093/ibd/izz017
|
[39] |
O’Sullivan SE. Endocannabinoids and the cardiovascular system in health and disease[M]//Pertwee RG. Endocannabinoids. Cham: Springer, 2015: 393–422.
|
[40] |
Di Paola R, Cordaro M, Crupi R, et al. Protective effects of ultramicronized palmitoylethanolamide (PEA-um) in myocardial ischaemia and reperfusion injury in vivo[J]. Shock, 2016, 46(2): 202–213. doi: 10.1097/SHK.0000000000000578
|
[41] |
Gugliandolo E, Fusco R, Biundo F, et al. Palmitoylethanolamide and polydatin combination reduces inflammation and oxidative stress in vascular injury[J]. Pharmacol Res, 2017, 123: 83–92. doi: 10.1016/j.phrs.2017.06.014
|
[42] |
D’Amico R, Fusco R, Gugliandolo E, et al. Effects of a new compound containing palmitoylethanolamide and baicalein in myocardial ischaemia/reperfusion injury in vivo[J]. Phytomedicine, 2019, 54: 27–42. doi: 10.1016/j.phymed.2018.09.191
|
[43] |
D’Amico R, Monaco F, Siracusa R, et al. Ultramicronized palmitoylethanolamide in the management of sepsis-induced coagulopathy and disseminated intravascular coagulation[J]. Int J Mol Sci, 2021, 22(21): 11388. doi: 10.3390/ijms222111388
|
[44] |
Raso GM, Pirozzi C, di Villa Bianca RD, et al. Palmitoylethanolamide treatment reduces blood pressure in spontaneously hypertensive rats: involvement of cytochrome p450-derived eicosanoids and renin angiotensin system[J]. PLoS One, 2015, 10(5): e0123602. doi: 10.1371/journal.pone.0123602
|
[45] |
Marichal-Cancino BA, González-Hernández A, MaassenVanDenBrink A, et al. Potential mechanisms involved in palmitoylethanolamide-induced vasodepressor effects in rats[J]. J Vasc Res, 2020, 57(3): 152–163. doi: 10.1159/000506158
|
[46] |
Chua JT, Argueta DA, DiPatrizio NV, et al. Endocannabinoid system and the kidneys: from renal physiology to injury and disease[J]. Cannabis Cannabinoid Res, 2019, 4(1): 10–20. doi: 10.1089/can.2018.0060
|
[47] |
Impellizzeri D, Bruschetta G, Ahmad A, et al. Effects of palmitoylethanolamide and silymarin combination treatment in an animal model of kidney ischemia and reperfusion[J]. Eur J Pharmacol, 2015, 762: 136–149. doi: 10.1016/j.ejphar.2015.05.010
|
[48] |
Raso GM, Simeoli R, Russo R, et al. N-Palmitoylethanolamide protects the kidney from hypertensive injury in spontaneously hypertensive rats via inhibition of oxidative stress[J]. Pharmacol Res, 2013, 76: 67–76. doi: 10.1016/j.phrs.2013.07.007
|
[49] |
Samsu N. Diabetic nephropathy: challenges in pathogenesis, diagnosis, and treatment[J]. Biomed Res Int, 2021, 2021: 1497449. doi: 10.1155/2021/1497449
|
[50] |
Wada J, Makino H. Inflammation and the pathogenesis of diabetic nephropathy[J]. Clin Sci, 2013, 124(3): 139–152. doi: 10.1042/CS20120198
|
[51] |
Impellizzeri D, Esposito E, Attley J, et al. Targeting inflammation: new therapeutic approaches in chronic kidney disease (CKD)[J]. Pharmacol Res, 2014, 81: 91–102. doi: 10.1016/j.phrs.2014.02.007
|
[52] |
Cordaro M, Impellizzeri D, Bruschetta G, et al. A novel protective formulation of palmitoylethanolamide in experimental model of contrast agent induced nephropathy[J]. Toxicol Lett, 2016, 240(1): 10–21. doi: 10.1016/j.toxlet.2015.10.006
|
[53] |
Sacco RL, Kasner SE, Broderick JP, et al. An updated definition of stroke for the 21st century: a statement for healthcare professionals from the American Heart Association/American Stroke Association[J]. Stroke, 2013, 44(7): 2064–2089. doi: 10.1161/STR.0b013e318296aeca
|
[54] |
Jin R, Yang G, Li G. Molecular insights and therapeutic targets for blood-brain barrier disruption in ischemic stroke: critical role of matrix metalloproteinases and tissue-type plasminogen activator[J]. Neurobiol Dis, 2010, 38(3): 376–385. doi: 10.1016/j.nbd.2010.03.008
|
[55] |
Ahmad A, Genovese T, Impellizzeri D, et al. Reduction of ischemic brain injury by administration of palmitoylethanolamide after transient middle cerebral artery occlusion in rats[J]. Brain Res, 2012, 1477: 45–58. doi: 10.1016/j.brainres.2012.08.006
|
[56] |
Kong D, Xie B, Li Y, et al. PEA prevented early BBB disruption after cerebral ischaemic/reperfusion (I/R) injury through regulation of ROCK/MLC signaling[J]. Biochem Biophys Res Commun, 2021, 566: 164–169. doi: 10.1016/j.bbrc.2021.06.019
|
[57] |
Lu W, Chen Z, Wen J. The role of RhoA/ROCK pathway in the ischemic stroke-induced neuroinflammation[J]. Biomed Pharmacother, 2023, 165: 115141. doi: 10.1016/j.biopha.2023.115141
|
[58] |
Zhou G, Fu X, Wang L, et al. Palmitoylethanolamide ameliorates neuroinflammation via modulating PPAR-α to promote the functional outcome after intracerebral hemorrhage[J]. Neurosci Lett, 2022, 781: 136648. doi: 10.1016/j.neulet.2022.136648
|
[59] |
Caltagirone C, Cisari C, Schievano C, et al. Co-ultramicronized palmitoylethanolamide/luteolin in the treatment of cerebral ischemia: from rodent to man[J]. Transl Stroke Res, 2016, 7(1): 54–69. doi: 10.1007/s12975-015-0440-8
|
[60] |
Bonzanino M, Riolo M, Battaglini I, et al. PEALut in the dietary management of patients with acute ischemic stroke: a prospective randomized controlled clinical trial[J]. J Clin Med, 2024, 13(2): 509. doi: 10.3390/jcm13020509
|
[61] |
Biscetti L, Cresta E, Cupini LM, et al. The putative role of neuroinflammation in the complex pathophysiology of migraine: from bench to bedside[J]. Neurobiol Dis, 2023, 180: 106072. doi: 10.1016/j.nbd.2023.106072
|
[62] |
Greco R, Demartini C, Zanaboni AM, et al. Endocannabinoid system and migraine pain: an update[J]. Front Neurosci, 2018, 12: 172. doi: 10.3389/fnins.2018.00172
|
[63] |
Papetti L, Sforza G, Tullo G, et al. Tolerability of palmitoylethanolamide in a pediatric population suffering from migraine: a pilot study[J]. Pain Res Manag, 2020, 2020: 3938640. doi: 10.1155/2020/3938640
|
[64] |
Chirchiglia D, Cione E, Caroleo MC, et al. Effects of add-on ultramicronized N-palmitol ethanol amide in patients suffering of migraine with aura: a pilot study[J]. Front Neurol, 2018, 9: 674. doi: 10.3389/fneur.2018.00674
|
[65] |
Hernández AG. Palmitoylethanolamide-based nutraceutical Calmux® in preventive treatment of migraine[J]. Clin Neurol Neurosurg, 2022, 218: 107282. doi: 10.1016/j.clineuro.2022.107282
|
[66] |
Lo Castro F, Baraldi C, Pellesi L, et al. Clinical evidence of cannabinoids in migraine: a narrative review[J]. J Clin Med, 2022, 11(6): 1479. doi: 10.3390/jcm11061479
|
[67] |
Landolfo E, Cutuli D, Petrosini L, et al. Effects of palmitoylethanolamide on neurodegenerative diseases: a review from rodents to humans[J]. Biomolecules, 2022, 12(5): 667. doi: 10.3390/biom12050667
|
[68] |
Calabrò RS, Naro A, De Luca R, et al. PEALut efficacy in mild cognitive impairment: evidence from a SPECT case study![J]. Aging Clin Exp Res, 2016, 28(6): 1279–1282. doi: 10.1007/s40520-016-0533-6
|
[69] |
Facchinetti R, Valenza M, Gomiero C, et al. Co-ultramicronized palmitoylethanolamide/luteolin restores oligodendrocyte homeostasis via peroxisome proliferator-activated receptor-α in an in vitro model of Alzheimer’s disease[J]. Biomedicines, 2022, 10(6): 1236. doi: 10.3390/biomedicines10061236
|
[70] |
Çınar E, Tel BC, Şahin G. Neuroinflammation in Parkinson’s disease and its treatment opportunities[J]. Balkan Med J, 2022, 39(5): 318–333. doi: 10.4274/balkanmedj.galenos.2022.2022-7-100
|
[71] |
Brotini S, Schievano C, Guidi L. Ultra-micronized palmitoylethanolamide: an efficacious adjuvant therapy for Parkinson’s disease[J]. CNS Neurol Disord Drug Targets, 2017, 16(6): 705–713. doi: 10.2174/1871527316666170321124949
|
[72] |
Brotini S. Palmitoylethanolamide/Luteolin as adjuvant therapy to improve an unusual case of camptocormia in a patient with Parkinson’s disease: a case report[J]. Innov Clin Neurosci, 2021, 18(10-12): 12–14. https://pubmed.ncbi.nlm.nih.gov/35096476/
|
[73] |
Sari Y. Huntington’s disease: from mutant huntingtin protein to neurotrophic factor therapy[J]. Int J Biomed Sci, 2011, 7(2): 89–100. doi: 10.59566/IJBS.2011.7089
|
[74] |
Roos RAC. Huntington’s disease: a clinical review[J]. Orphanet J Rare Dis, 2010, 5: 40. doi: 10.1186/1750-1172-5-40
|
[75] |
Möller T. Neuroinflammation in Huntington’s disease[J]. J Neural Transm, 2010, 117(8): 1001–1008. doi: 10.1007/s00702-010-0430-7
|
[76] |
Meade E, Garvey M. The role of neuro-immune interaction in chronic pain conditions; functional somatic syndrome, neurogenic inflammation, and peripheral neuropathy[J]. Int J Mol Sci, 2022, 23(15): 8574. doi: 10.3390/ijms23158574
|
[77] |
Di Cesare Mannelli L, D’Agostino G, Pacini A, et al. Palmitoylethanolamide is a disease-modifying agent in peripheral neuropathy: pain relief and neuroprotection share a PPAR-alpha-mediated mechanism[J]. Mediators Inflamm, 2013, 2013: 328797. doi: 10.1155/2013/328797
|
[78] |
Desai K, Madan M, Sorathia Z, et al. FibrosetTM and neuromuscular pain: a multicentric, real world, observational, post-marketing surveillance study in Indian patients suffering from neuromuscular pain[J]. Int J Res Orthop, 2023, 9(5): 1009–1015. doi: 10.18203/issn.2455-4510.IntJResOrthop20232615
|
[79] |
Feldman EL, Goutman SA, Petri S, et al. Amyotrophic lateral sclerosis[J]. Lancet, 2022, 400(10360): 1363–1380. doi: 10.1016/S0140-6736(22)01272-7
|
[80] |
Dobson R, Giovannoni G. Multiple sclerosis - a review[J]. Eur J Neurol, 2019, 26(1): 27–40. doi: 10.1111/ene.13819
|
[81] |
Orefice NS, Alhouayek M, Carotenuto A, et al. Oral palmitoylethanolamide treatment is associated with reduced cutaneous adverse effects of interferon-β1a and circulating proinflammatory cytokines in relapsing-remitting multiple sclerosis[J]. Neurotherapeutics, 2016, 13(2): 428–438. doi: 10.1007/s13311-016-0420-z
|
[82] |
Clauw DJ. Fibromyalgia: a clinical review[J]. JAMA, 2014, 311(15): 1547–1555. doi: 10.1001/jama.2014.3266
|
[83] |
Bains A, Kohrman S, Punko D, et al. A link between inflammatory mechanisms and fibromyalgia[M]//Kim YK. Neuroinflammation, Gut-Brain Axis and Immunity in Neuropsychiatric Disorders. Singapore: Springer, 2023: 357–378.
|
[84] |
Del Giorno R, Skaper S, Paladini A, et al. Palmitoylethanolamide in fibromyalgia: results from prospective and retrospective observational studies[J]. Pain Ther, 2015, 4(2): 169–178. doi: 10.1007/s40122-015-0038-6
|
[85] |
Salaffi F, Farah S, Sarzi-Puttini P, et al. Palmitoylethanolamide and acetyl-L-carnitine act synergistically with duloxetine and pregabalin in fibromyalgia: results of a randomised controlled study[J]. Clin Exp Rheumatol, 2023, 41(6): 1323–1331. https://www.clinexprheumatol.org/abstract.asp?a=19342
|
[86] |
Schweiger V, Martini A, Bellamoli P, et al. Ultramicronized palmitoylethanolamide (um-PEA) as add-on treatment in fibromyalgia syndrome (FMS): retrospective observational study on 407 patients[J]. CNS Neurol Disord Drug Targets, 2019, 18(4): 326–333. doi: 10.2174/1871527318666190227205359
|
[87] |
Schwitzer T, Schwan R, Angioi-Duprez K, et al. The endocannabinoid system in the retina: from physiology to practical and therapeutic applications[J]. Neural Plast, 2016, 2016: 2916732. doi: 10.1155/2016/2916732
|
[88] |
Kaur G, Singh NK. Inflammation and retinal degenerative diseases[J]. Neural Regen Res, 2023, 18(3): 513–518. doi: 10.4103/1673-5374.350192
|
[89] |
Hesselink JMK, Costagliola C, Fakhry J, et al. Palmitoylethanolamide, a natural retinoprotectant: its putative relevance for the treatment of glaucoma and diabetic retinopathy[J]. J Ophthalmol, 2015, 2015: 430596. doi: 10.1155/2015/430596
|
[90] |
Chen J, Matias I, Dinh T, et al. Finding of endocannabinoids in human eye tissues: implications for glaucoma[J]. Biochem Biophys Res Commun, 2005, 330(4): 1062–1067. doi: 10.1016/j.bbrc.2005.03.095
|
[91] |
Gagliano C, Ortisi E, Pulvirenti L, et al. Ocular hypotensive effect of oral palmitoyl-ethanolamide: a clinical trial[J]. Invest Ophthalmol Vis Sci, 2011, 52(9): 6096–6100. doi: 10.1167/iovs.10-7057
|
[92] |
Strobbe E, Cellini M, Campos EC. Effectiveness of palmitoylethanolamide on endothelial dysfunction in ocular hypertensive patients: a randomized, placebo-controlled cross-over study[J]. Invest Ophthalmol Vis Sci, 2013, 54(2): 968–973. doi: 10.1167/iovs.12-10899
|
[93] |
Costagliola C, Romano MR, dell’Omo R, et al. Effect of palmitoylethanolamide on visual field damage progression in normal tension glaucoma patients: results of an open-label six-month follow-up[J]. J Med Food, 2014, 17(9): 949–954. doi: 10.1089/jmf.2013.0165
|
[94] |
Rossi GCM, Scudeller L, Lumini C, et al. Effect of palmitoylethanolamide on inner retinal function in glaucoma: a randomized, single blind, crossover, clinical trial by pattern-electroretinogram[J]. Sci Rep, 2020, 10(1): 10468. doi: 10.1038/s41598-020-67527-z
|
[95] |
Tan TE, Wong TY. Diabetic retinopathy: looking forward to 2030[J]. Front Endocrinol, 2023, 13: 1077669. doi: 10.3389/fendo.2022.1077669
|
[96] |
Paterniti I, Di Paola R, Campolo M, et al. Palmitoylethanolamide treatment reduces retinal inflammation in streptozotocin-induced diabetic rats[J]. Eur J Pharmacol, 2015, 769: 313–323. doi: 10.1016/j.ejphar.2015.11.035
|
[97] |
Thomas CJ, Mirza RG, Gill MK. Age-related macular degeneration[J]. Med Clin North Am, 2021, 105(3): 473–491. doi: 10.1016/j.mcna.2021.01.003
|
[98] |
Tan W, Zou J, Yoshida S, et al. The role of inflammation in age-related macular degeneration[J]. Int J Biol Sci, 2020, 16(15): 2989–3001. doi: 10.7150/ijbs.49890
|
[99] |
Ye S, Chen Q, Jiang N, et al. PPARα-dependent effects of palmitoylethanolamide against retinal neovascularization and fibrosis[J]. Invest Ophthalmol Vis Sci, 2020, 61(4): 15. doi: 10.1167/iovs.61.4.15
|
[100] |
Fraher D, Mann RJ, Dubuisson MJ, et al. The endocannabinoid system and retinoic acid signaling combine to influence bone growth[J]. Mol Cell Endocrinol, 2021, 529: 111267. doi: 10.1016/j.mce.2021.111267
|
[101] |
Briskey D, Roche G, Rao A. The effect of a dispersible palmitoylethanolamide (Levagen+) compared to a placebo for reducing joint pain in an adult population – a randomised, double-blind study[J]. Int J Nutr Food Sci, 2021, 10(1): 9–13. doi: 10.11648/j.ijnfs.20211001.12
|
[102] |
Jung JI, Lee HS, Jeon YE, et al. Anti-inflammatory activity of palmitoylethanolamide ameliorates osteoarthritis induced by monosodium iodoacetate in Sprague-Dawley rats[J]. Inflammopharmacology, 2021, 29(5): 1475–1486. doi: 10.1007/s10787-021-00870-3
|
[103] |
De Petrocellis L, Melck D, Bisogno T, et al. Endocannabinoids and fatty acid amides in cancer, inflammation and related disorders[J]. Chem Phys Lipids, 2000, 108(1-2): 191–209. doi: 10.1016/S0009-3084(00)00196-1
|
[104] |
Di Marzo V, Melck D, Orlando P, et al. Palmitoylethanolamide inhibits the expression of fatty acid amide hydrolase and enhances the anti-proliferative effect of anandamide in human breast cancer cells[J]. Biochem J, 2001, 358(Pt 1): 249–255.
|
[105] |
Molloy NH, Read DE, Gorman AM. Nerve growth factor in cancer cell death and survival[J]. Cancers (Basel), 2011, 3(1): 510–530. doi: 10.3390/cancers3010510
|
[106] |
Giovannucci E. Modifiable risk factors for colon cancer[J]. Gastroenterol Clin North Am, 2002, 31(4): 925–943. doi: 10.1016/S0889-8553(02)00057-2
|
[107] |
Pagano E, Venneri T, Lucariello G, et al. Palmitoylethanolamide reduces colon cancer cell proliferation and migration, influences tumor cell cycle and exerts in vivo chemopreventive effects[J]. Cancers (Basel), 2021, 13(8): 1923. doi: 10.3390/cancers13081923
|
[108] |
Johnson CA, James D, Marzan A, et al. Cervical cancer: an overview of pathophysiology and management[J]. Semin Oncol Nurs, 2019, 35(2): 166–174. doi: 10.1016/j.soncn.2019.02.003
|
[109] |
Anchoori RK, Khan SR, Sueblinvong T, et al. Stressing the ubiquitin-proteasome system without 20S proteolytic inhibition selectively kills cervical cancer cells[J]. PLoS One, 2011, 6(8): e23888. doi: 10.1371/journal.pone.0023888
|
[110] |
Tu Y, Chen C, Pan J, et al. The ubiquitin proteasome pathway (UPP) in the regulation of cell cycle control and DNA damage repair and its implication in tumorigenesis[J]. Int J Clin Exp Pathol, 2012, 5(8): 726–738. https://pubmed.ncbi.nlm.nih.gov/23071855/
|
[111] |
Bonfili L, Cecarini V, Eleuteri AM. Antiproliferative effects of palmitoylethanolamide on human cervical cancer cells[C]//International Conference on Cancer Research 2019. London, UK, 2019: 154–162. (查阅网上资料, 未找到本条文献出版者信息, 请确认)
|
[112] |
Di Stadio A, Gallina S, Cocuzza S, et al. Treatment of COVID-19 olfactory dysfunction with olfactory training, palmitoylethanolamide with luteolin, or combined therapy: a blinded controlled multicenter randomized trial[J]. Eur Arch Otorhinolaryngol, 2023, 280(11): 4949–4961. doi: 10.1007/s00405-023-08085-8
|
[113] |
Marini I, Bartolucci ML, Bortolotti F, et al. Palmitoylethanolamide versus a nonsteroidal anti-inflammatory drug in the treatment of temporomandibular joint inflammatory pain[J]. J Orofac Pain, 2012, 26(2): 99–104. https://pubmed.ncbi.nlm.nih.gov/22558609/
|
[114] |
Cruccu G, Di Stefano G, Marchettini P, et al. Micronized palmitoylethanolamide: a post hoc analysis of a controlled study in patients with low back pain – sciatica[J]. CNS Neurol Disord Drug Targets, 2019, 18(6): 491–495. doi: 10.2174/1871527318666190703110036
|